The Magic of Ceramics
The spark that really advanced ceramic technology and contributed the next important pieces to the modern ceramics puzzle was the invention of the automobile. Early automobiles, such as those developed by Daimler and Benz in Germany in the s, needed a simple way to ignite the tiny fire that powered their internal combustion engines, much as a match needs to be struck against a special surface to start it burning.
Spark plugs provided that magical flash of fire. A spark plug consists of a ceramic electrical insulator shaped like a tube, with a metal pin inside it and a separate metal case partly surrounding From Pottery to the Space Shuffle it. The metal case contains a hook that bends around the pin to form what is called an air gap. The small explosion that results pushes a piston and provides mechanical power to the engine. These actions create a really severe environment for a ceramic to survive. Early s glass and ceramics could not reliably survive these severe conditions.
In those early days of the automobile, driving 50 miles was a great adventure, usually resulting in at least one breakdown to replace a spark plug. Extensive research and development were conducted between about and to achieve ceramics that provided longer life and durability in both automotive and aircraft spark plugs. This laid the groundwork for the rapid emergence of modern ceramics. It involved incremental changes in the spark plug insulator composition from a traditional porcelain made with naturally occurring raw materials to high-alumina compositions made with chemically synthesized powders.
The transition sounds easy, but it actually required entirely new technologies in powder synthesis, shape fabrication, fabrication equipment, and manufacturing scale-up. The porcelain was fabricated from the naturally occurring raw materials clay, flint, and feldspar. These spark plugs were not reliable. As alumina powder was added and clay deleted, however, the mixture no longer became pliable with the addition of water, so traditional pottery-shaping techniques did not work. Alternate techniques were developed, through which the shape was achieved by adding an organic binder glue to the powder and compacting the mixture under pressure inside a shaped cavity, a method similar to the dust-pressing technique developed for tile in England.
The powder compact then could be removed from the cavity, machined to a final shape, and fired in a furnace to remove the organic Figure Automotive spark plug showing some of the metal parts and the white alumina Ceramic in5UkItOr. As the years passed, these new processes were refined, and even automated, to meet the high production rate required for automobiles and aircraft.
Even by , large quantities of spark plugs were being fabricated. AC Spark Plug, for example, reported producing about 60, spark plugs per day just for World War I aircraft requirements. By , over million spark plugs were produced per year worldwide, and by , more than 1billion per year rolled off the assembly lines worldwide. Achieving high-temperature furnaces mentioned earlier, was one of the challenges that had to be overcome in spark plug development.
However, as the percent of alumina increased, the temperature requirement went beyond the capability of existing production furnaces. New technology was successfully developed to design and build production furnaces to reach these temperatures, adding another key piece to the puzzle. The final piece of the puzzle was to achieve a method that could produce, at low cost, thousands of tons of pure ceramic powders such as alumina. Large-scale chemical production of alumina powder had been developed by Bayer the same company that introduced aspirin clear back in , to supply aluminum oxide raw material for aluminum metal production, but the Bayer powder contained too many sodium impurities to be useful for the new ceramics, Finally, by about , scientists succeeded in producing highpurity, low-cost alumina powder on a large scale.
Alumina Spreads to Other Modern Applications Once the powder synthesis, composition, and fabrication challenges were solved, high-alumina ceramics became the standard for spark plugs that last for thousands of hours and are one of the most reliable products we can buy. Besides their superior electrical-resistancebehavior, high-alumina ceramics also proved to be more resistant to wear, erosion, and corrosion than traditional ceramics.
This overall superiority led to a wide variety of products, including abrasives and grinding wheels; wear-resistant tiles for industrial and mining equipment; labware resistant to acids and bases; seals; pump parts; and even bullet-resistant armor. Alumina has been so from Pottery to the Space Shuffle successful that production reached a level of approximately five million metric tons per year by , enough to make a solid block the size of a football field and nearly feet high.
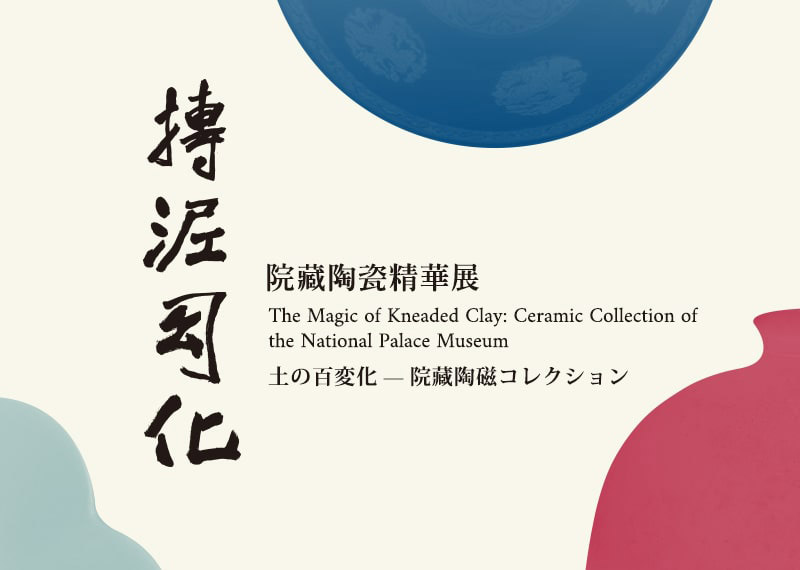
The Explosion of New Ceramics and Ceramic Uses Alumina was the pioneer of advanced ceramics, the model to be emulated for numerous other advanced ceramic materials that followed. The lessons ceramists had learned from creating products with alumina ceramics were applied to engineering all of the later ceramic compositions. Whole families of ceramics were invented: In the past years alone, our knowledge of ceramic materials has exploded. We now understand some of the behavior that baffled mankind for centuries.
We can design and engineer ceramics, and combine ceramics with other materials, to solve just about any problem or challenge we encounter. We owe a debt of gratitude to the new advanced, or engineered, ceramics for many of the remarkable products that we now take so much for granted-products such as radios, color televisions, fiberglass, lasers, ultrasonics, microwave and fiberoptic communications devices, advanced jet aircraft, home computers, and cellular phones.
The exact date and circumstances of the first piece of "ceramic art" are not known, but archaeological discoveries suggest that it was a vessel or figurine hand-formed from damp clay and hardened in the sun. Such items probably were not intended as a r t but instead as religious articles to assure fertility or successful hunting. Potters decorated this pottery after it had been fired by painting on simple designs with a reddish pigment over a lighter colored clay slip.
The pigment and slip were made by mixing the selected color of powdered clay with water to turn the mixtures into thin pastes that could then be smeared or painted onto the surface of the porous got. Some of the clay particles soaked into the pores with the water, allowing the clay decoration to adhere to the pot. The decorations were geometric patterns, similar to cave wall paintings from the same region. Decorated pottery also has been found at many sites in ancient Mesopotamia and Egypt estimated to date back to roughly to BC.
Mesopotamia extended along the valleys of the Tigris and Euphrates Rivers from the east end of the Mediterranean Sea to the Persian Gulf and has sometimes been referred to as the Cradle of Civilization. Egypt was another important early center of civilization along the fertile flood plains of the Nile River.
The people from these regions, too, made clay-based earthenware, which t hey clay painted with simple designs a nd, occasionally, with animal and human figures. They also used a technique Figure 3- 7. One of the animal figures depicted in glazed tile on the lshtar Gate, which was constructed in Babylon around BC under the rule of Nebuchadnezzar For further variation, potters could apply their clay paint into the incised design.
If you were to look at some of these old pots in a museum and compare them to the simple, undecorated containers from much earlier years, you would see beauty evolving in the new earthenware. A big step in the evolution of beauty in pottery coincided with the dawn of the Bronze Age, around to BC. As discussed in Chapter 2, bronze could be formed into delicate, thin, contoured shapes.
Potters tried to mimic these shapes, opening up new options for beauty in their creations. New kilns that could fire the earthenware at higher temperature were a big help because of the resulting increased strength and decreased porosity. The new two-chamber kilns also opened up more options for decorations. Previous single-chamber kilns had caused any decorations applied before firing to wash out or fade. With the two-chamber kilns, the pots could be isolated in a separate compartment away.
Clay pigments could now be painted on before firing and would retain their color and sharp edge definition of designs, resulting in increased contrast between the pot and the decoration. This contrast made the decorations more visible and dramatic. The next spectacular additions to ceramic beauty were glazes and faience, followed by the creation of whole articles crafted from glass.
As also discussed in Chapter 2, early glazes emerged from efforts dating back to around BC to emulate the precious blue stone lapis lazuli. Special glazes containing lead were developed sometime between and BC in Babylon. The lead acted as a flux, to allow the glaze to form at a lower temperature. Pigments that lost their color at higher temperatures could now be used, resulting in brighter colors and a larger variety of colors. Lead glazes could be applied over the prefired ceramic or even over a highertemperature glaze, opening up a whole new realm of artistic creativity.
By the 8th century BC,another glaze additive was discovered, this time by the Assyrians in Persia. This additive, tin oxide, yielded an opaque white glaze that could actually hide the underlying brown or reddish clay earthenware, so that the ceramic piece appeared much lighter in color.
Glazes could now really show off their true colors! This 7 7 inch high vase is from to BC and was probably used as a storage jar. The side shown depicts a mythical combat scene. The other side depicts Apollo, the god of light and music, standing between his mother and sister, playing a kithara.
The Ishtar Gate was about The most important animal figure was probably Musrussu the dragon, which served the chief Babylonian god Marduk Baal. Musrussu was portrayed with the body and head of a serpent, the front legs of a lion, the hind legs and talons of an eagle, and the neck and mane of a bull. Greek and Roman Ceramics The rise of Greek civilization around BC had a favorable influence on all of the arts, including ceramics. The Greeks believed strongly that community, religion, and art were closely linked, and art was an integral Beauty of Ceramics e part of their daily lives.
Greek pottery richly depicted scenes of daily life and mythology. The pots were wheel-thrown and painted with a slip containing very fine particles of clay. Additional detail was achieved by scratching through the painted image to reveal the color of the underlying earthenware. Early pots about to BC had black scenes bordered with decorative geometric and floral designs, all on a red or yellowish background. Later pots about to BC presented red figures with other colors on a black background. Each of these Greek styles illustrates a story in a dramatic contrast of colors.
Interestingly, the Greek potters chose not to use glazes. By BC,the classical Greek civilization had begun to decline and was eventually absorbed into Roman civilization. The Romans gleaned ideas and technology from many cultures and spread them widely. One of the most imporFigure 3 Early and colors of glass vessels produced by early glass blowers.
They blew glass vessels in numerous shapes, sizes and colors. Some vessels were blown into the open air and either took a natural free-form shape or were contoured with ceramic or metallic tools, while still hot and soft, into other creative forms. Others were blown into shaped molds, so that the glass bubble deformed to duplicate the shape of the inside of the mold. Glass molding opened a wide range of creative opportunities. Some artisans engraved inside the molds elaborate scenes, which were then embossed into the glass during blowing.
Ceramics in the Far East China was another early center of civilization. Just as i n t h e Mediterranean region, the earliest ceramics were earthenware, and the earliest decorations were painted on with clay or iron oxide rust pigments. Potters formed the moistened clay into coils and stacked the coils in rows on top of a flat, circular clay base. The surface of the pot was then rubbed burnished with a smooth tool, giving the ceramic piece a smooth, polished surface before firing.
Variants of this technique are still used today by artisans in many parts of the world. Early Chinese pots were adorned with spirals, checkered and dotted patterns, fish, frogs, deer, and snakes, but very rarely with human faces. The decorations probably had specific religious or ritual meanings, because many of the early Chinese pots have been found in burial sites, although some decorated urns also have been found at ancient home sites. This pottery seems to have been prepared using a fastmoving wheel and was similar in color, shape, and decoration to earlier black pottery from several Mediterranean regions.
Longshan pottery was exquisitely refined and eggshell thin. Firing under reducing conditions is still commonly done today to obtain special visual effects. Neolithic Chinese storage jar. Painted earthenware jar, 7 7 inches high, from the Yangshao culture, Banshan cultural phase of Cansu Province. Coil-built with buff clay, covered with orange slip, and decorated with black pigment. Decorations limited to the upper portion because the lower portion was designed to be placed firmly into the ground. High-temperature kilns, special clays such as kaolin, and high-temperature glazes led the Chinese potters along a different path from that followed by Western potters, resulting in an incredible legacy of innovations and ceramic creations unique to the East.
The Chinese developed and refined stoneware and porcelain and centuries later exported these ceramics to other countries. On the other hand, they imported glass and lead glaze technologies. I Ceramics in the Americas The earliest pottery in the Americas was found in Ecuador and is estimated to have originated around BC.
Seated figure from Colima, Mexico. Fourteen inch earthenware figure from about AD , found in a shaft tomb in western Mexico. Pottery figures were commonly buried with the dead as shamans priests to protect from evil spirits or as companions to participate in the activities of the afterlife. Before that, all of the American pottery was earthenware shaped by hand, decorated with slips, and fired in rather simple kilns. The potter's wheel was never developed in the Americas, and glazes were relatively unknown.
In spite of their limited technology, ancient Americans managed to create highly distinctive decorations, as well as numerous shapes and styles of pottery. For example, the absence of the potter's wheel led them to a greater variety of imaginative, free-form a n d noncircular shapes t h a n were produced by early Mediterranean and Chinese potters. A popular style was hand-sculpted containers shaped like animals whose heads served as pouring spouts and 4 Figure The frame of beads around the face represents a cave, indicating that the god is in the underworld.
Earthenware with chestnut and three-color glazes, this Courtesy of the Utah Museum of Fine Arts. Photograph by lim Frankoski. Gift to the museum from Professor and Mn. Lennox Tierney and the Friends of the Art Museum. Another imaginative style of container had a hollow stirrup-shaped carrying handle arching [ over the top, with a pouring spout built into the handle. Other earthenware creations were in the images of important gods.
Most of the ceramic works were decorated with highly stylized animals, insects, persons, gods, and geometric designs, usually painted in a dark color on a light background. These years made up the period we now refer to as the Dark Ages or Middle Ages. Some stagnation also occurred in other areas of the world between about AD and Even China had an unsettled period between the Han Dynasty which ended in AD and the Tang Dynasty which started in AD , although stoneware and glazes continued to improve.
The Tang Dynasty began many centuries of reasonable stability in China that encouraged the refinement of ceramics. About the same time, Islam arose in the Middle East, leading to a period of stability that also encouraged innovations and creativity in ceramics. Chinese Ceramics during the Middle Ages The Tang Dynasty in China, strongly influenced by the spread of Buddhism from India, was a period of peace, tolerance, and substantial interaction and trade with other cultures-a time of great economic prosperity and wealth.
It was a perfect environment for ceramic creativity to flourish. Incredibly beautiful ceramic items were crafted, but they were not necessarily considered art at the time by the Chinese. These creations generally had a deeper, spiritual meaning that was an integral part of the Chinese philosophy of life. Today, however, we value Chinese works as art treasures.
Some of the most spectacular ceramic items during the Tang Dynasty were commissioned by wealthy aristocrats. Their purpose was to Beauty of Ceramics e Figure Commissioned for use in a Chinese imperial palace around AD 7 to , during the Qianlong period. Bats were a common theme. The Chinese words for bat and happiness coincide.
Vase from the collection of Mr. The camel was a popular subject, based on its importance to the silk trade. Most people have heard of the renowned Ming vases of China. These vases were made of fine white porcelain decorated with intricate 1 scenes and designs that looked almost as if they had been drawn I I on with ink. This slip was del! During firing, the cobalt oxide turned the ink a permanent blue, and the glaze became a bright, colorless varnish to accent and protect the scene. Other inks were developed with different colors. Still other beautiful effects were achieved by painting with colored enamels.
Enamels were low-temperature glazes that were painted onto the smooth surface of a fired, high-temperature glaze and refired at a low temperature to produce scenes in a variety of colors. I 1 Other innovations in China included the development of slip casting during the Tang Dynasty and of copper-red coloration during the Ming Dynasty. Slip casting involved pouring a fluid slip a mixture of clay and other ceramic particles suspended in water, similar to the early clay paints into a porous mold. Water was sucked out of the slip into the pores of the mold, and the ceramic particles in the slip were deposited on the inner walls of the mold.
When the desired wall thickness was achieved, the remaining slip was poured out of the mold. After partial drying, the ceramic ware could be removed from the mold, decorated, and fired. The mold was made of bisque-fired ceramic-ceramic fired at a lower temperature than normal, so that it had high porosity. Slip casting did not become a popular fabrication method in China, but centuries later, it was important for the fabrication of European porcelain, and it is widely used today for everything from figurines and beer mugs to sinks and toilets.
The second innovation, copper-red color, was achieved by applying a thin wash of copper oxide over a glaze and firing under reducing low-oxygen conditions. A whole range of colors, from salmon to pink to blood red, could be produced with that tecnhique. The chip was mounted onto a glass-fiber-reinforced plastic strip, connected by tiny wires to conductive metal circuits, and potted enclosed in a protective layer of plastic.
Part of the plastic has been cut away to reveal a portion of the chip. The battery and liquid crystal display, both containing ceramic materials, also are shown. By the early s, silicon chips one-tenth of an inch square each contained thousands of electrical components and were complex enough to be the brain inside a hand-held calculator.
This electronic innovation became known as large-scale integration LSI. From then on, technology progressed at breathtaking speed. Very large-scale integration VLSI , with more than 3 million transistors on a silicon chip, was introduced in the s. Such a chip, no larger than your thumbnail, could be designed with all of the electrical circuits necessary for a computer that previously had filled a whole room.
Now chips with 16 million transistors are commonplace. They start by making each device incredibly tiny, about one twenty-millionth of an inch across about nanometers. These devices are built up on the surface of a slice of singlecrystal silicon, layer after layer. A single chip can have more than 20 layers, all interconnected like a three-dimensional maze.
To imagine the complexity of an IC, think of a 20 story building filled with thousands of apartments. Each apartment is full of electrical wiring and different appliances such as refrigerators, toasters, and hair dryers. These appliances are like the individual devices in the microchip, each requiring its own special electrical connections and input, yet all interconnected to the main electrical wiring of the whole building.
Now, imagine the network of pipes aad plumbing for distributing water; this is like another network of devices, forming a separate circuit but also necessary to the overall duties of the integrated circuit. Finally, imagine all of the hallways and doors. These represent another circuit.
Now shrink all of the electrical wiring, plumbing, halls, electrical switches, faucets, and doors in our 20 story building down to the size of a fingernail, but squashed and compacted to leave n o open spaces. This incredibly compact structure is a pretty good illustration of the complexity of a modern IC. The electrical wiring, plumbing, and halls represent the complex electrical paths in the IC.
The electrical switches, faucets, and doors represent all of the microswitches and control gates that route the electrical current through the IC. The millions of appliances represent the individual electrical devices built into each IC. Engineers who design ICs select which devices to build in, according to the desired use of the IC: Most of the materials in an IC are semiconductors, meaning they can block electricity under some circumstances and conduct electricity under other circumstances, as discussed earlier.
They can act as tiny microswitches to route electricity through the IC to perform tasks such as storing information computer memory or going through a complex sequence of mathematical calculations calculator or computer. Some materials, such as silicon and germanium, are natural intrinsic semiconductors, because they have a narrow band gap; not much energy is needed for electrons to jump the energy gap and let electricity flow. This addition is called doping. Doping with boron makes extra electrons available in the silicon, so that electrical conduction can occur more easily by the flow of these extra negative charges.
Doping with phosphorus causes a deficiency in the number of electrons, which is the same as making positive charges electron holes available for conduction. By using natural and doped semiconductors, engineers can design just about any electrical behavior they want. However, they must also separate the different electrical circuits and devices from each other with an electrical insulator.
This separation is an important role for thin films, or layers, of ceramics.
- Rodents! Learn About Rodents and Enjoy Colorful Pictures - Look and Learn! (250+ Photos of Rodents)?
- Reward Yourself?
- www.newyorkethnicfood.com : The Magic of Ceramics () : David W. Richerson : Books?
- The Magic of Ceramics, 2nd Edition.
- Navigation;
- Bacon Bobs Big Book Of Bacon.
We identified three basic categories of materials at the start of this book: Into which of these categories do semiconductors used in ICs fit? Silicon, for example, is shiny and looks metallic, but the atoms are bonded together in exactly the same way as in diamond, which is usually used as one of the classical examples of a ceramic structure.
Germanium also has the same crystal structure as diamond. So, from an atomic-structure point of view, these semiconductor materials are more like ceramics. If we can reasonably consider these materials as ceramics, then most of an IC chip is made up of ceramics. Ceramics are involved in just about every step in making ICs. The following paragraphs describe briefly how an IC is fabricated and the role of ceramics.
The first step in IC fabrication is to produce a very pure, perfect single crystal of silicon. Remember our discussion in Chapter 2 on the growth Of ruby crysta1s by the Verneuil method, by dropping alumina powder through a high-temperature torch and building up a figure Two single-crystal silicon rods, showing 7 s fabrication capabilities; a large polished wafer about 8 inches in diameter, showing early s capability; and several chips mounted in ceramic packages.
Note the mirror polish on the large Wafer. Ceramics and the Electronics Age e single crystal boule? Once the silicon was melted, a single crystal of silicon was touched to the top surface of the melt, allowing silicon atoms from the melt to deposit. If the silicon crystal was very slowly pulled away from the molten silicon, a single crystal rod of silicon was produced, similar to the one shown in Figure In the early s, when ICs were first being developed, the largest single crystal of silicon that could be grown was about 1 inch in diameter.
The technology has come a long way. Now, highly perfect single crystals of silicon can be grown 12 inches in diameter and 40 inches long. Silicon carbide wafer boats above and a wafer boat loaded with silicon wafers and supported on a silicon carbide paddle below. The second step in IC fabrication is to slice the single-crystal rod into wafers about 25 thousandths of an inch thick and to grind a n d polish these wafers t o a n incredible degree of flatness and smoothness note the mirror finish of the wafer in Figure This shaping is necessary because the devices that will be formed later at and on the surface of the wafer are only 20 millionths of an inch nan0metel-s across.
Even the tiniest scratch Or pore disrupt the formation Of these devices. All the precision slicing, grinding, and polishing are done with ceramics. The polished silicon wafers are carefully cleaned to remove even the tiniest particle of dust or lint, loaded onto a ceramic carrier called a wafer boat, placed on a long-handled flat ceramic paddle, and inserted into a ceramic-lined furnace. The desired dopant to give the silicon wafer just the right semiconductor characteristic is also loaded into the furnace.
When the furnace is heated to the right temperature, the dopant diffuses soaks into the surface of the silicon. We now have the silicon base or substrate for our chip, but we still have to build the many layers of transistors and other devices and the ceramic electrical insulators separating them. This layering is done by repeatedly etching dissolving away a microscopic pattern of some of the silicon and depositing other ceramic materials in its place, layer by layer. The etching is done using a special process2known as photolithography. The silicon is coated with a light-sensitive polymer.
Light is projected through a lens and a mask a cutout pattern to shine the pattern of the particular layer of devices onto the silicon chip. The polymer struck by the light becomes sensitized, similarly to the Fotoform glass discussed in Chapter 4. The sensitized polymer and a tiny depth of silicon underneath can now be etched away by an acid. Since the devices must be accurate to about 20 millionths of an inch, the mask and lens must be very accurate. The lens, for example, is made of low-thermal-expansion fused Figure Vacuum chuck used to hold a silicon wafer in position during various high- temperature vapor deposition steps.
Approximately 12 inches across, showing outline of position of wafer. Photo courtesy of Saint-CobainKarborundum Structural The etching step is followed by a deposition silicon Ceramics, Niagara Falls, NY step, to fill the device material into the spaces etched out of the silicon.
These are all high-temperature processes that require ceramic furnace linings and support structures. The photolithography, etching, and deposition processes are repeated with different materials for each type of device in each layer, until all of the layers of the IC have been formed. This process is amazing and complex. Close-up of a s silicon wafer after deposition of devices, with the tip of a sewing pin for size COmpariSOn.
Any mistake made in any step ruins the whole wafer. Since each wafer contains hundreds of individual ICs, a ruined wafer is an expensive loss. A complete descriptiDn of the design and fabrication of ICs would take many pages and go into scientific details well beyond the scope of this book. Now What Happens with the Silicon Chip? Now that we have a silicon chip, what do we do with it? How is it mounted into a piece of electronics equipment?
We already saw one example in Figure The silicon chip was mounted onto a thin sheet of plastic reinforced with glass fibers. The chip next was connected by tiny wires to a pattern of metal circuits painted onto the plastic and leading to the battery, keyboard, and liquid-crystal display. A liquid polymer then was poured over the chip and wires and allowed to harden, just like an epoxy glue starts out as a liquid and then hardens: A silicon chip can be damaged by moisture in the air and so must be completely encapsulated.
Plastic encapsulation works fine for small ICs in calculators, but not for larger ICs in computers or other equipment that runs continuously. The ICs build up a lot of heat, enough to actually ruin the IC. Since plastic holds in heat, an alternate material must be used, one that can carry the heat away from the chip.
Aluminum oxide is the material most commonly used, but not only to protect the chip from moisture and carry away heat. A cross-section diagram of a hybrid package is shown in Figure Let's explore how Power Plane Grid this hybrid package is made and used in a mainframe computer. I- The hybrid package is made starting with alumina powder ground up as fine as dust. The powder is mixed with a liquid and a selected organic binder material sort of like white household glue that will Figure Schematic illustrating the construction of a hybrid multilayer ceramic become solid but flexible when package for mounting silicon chip integrated circuits From E.
The slip is poured onto a large, flat surface and spread out with a knife blade to a flat, thin layer and allowed to dry. After it's dried, the material is a mixture of ceramic particles and the flexible organic material and referred to as a tape.
- The Magic of Ceramics - PDF Free Download.
- Karyns Gift.
- Autobiography of a Dog.
- The Believer.
- The Magic of Ceramics.
- National Palace Museum?
- Join Kobo & start eReading today.
It looks a little like the very thin dough rolled out for making noodles or fine French pastry but is even thinner and very smooth on both surfaces. The next step is to cut the tape to the desired size a n d p u n c h t i n y holes where metal conductive paths through the layer are required. A paste of metal Figure Schematic showing the complexity of a 23 layer hybrid multilayer particles typically tungsten ceramic package. Courtesy of ISM, Hopewell Junction, NY or molybdenum mixed with an organic binder and a little liquid is printed onto the tape in the type of circuit patterns shown for the different layers in Figure This metal paste also fills the holes that were punched.
The different layers of tape, with their printed metal patterns, are now stacked and gently heated under slight pressure, to cause Ceramics and the Electronics Age w the organic binder in the tape to bond the layers of tape together. The large ICs for mainframe computers require more than 20 tape layers.
Web Content Display
The layers of tape are still just a bunch of ceramic and metal powder particles held together with an organic glue, just as if you mixed sand or dust with white glue. Before the hybrid package can be used for ICs, it must be converted to a solid ceramic interlaced with conductive metal channels. The hybrid IC package is a miracle of miniaturization that required many innovations in materials and fabrication technology.
A hybrid package built in the s could hold only one chip and provide the internal electrical wiring for only a few hundred devices. Examples of hybrid multilayer ceramic packages. Photos courtesy of Kyocera. Magnified view of a vintage 7 silicon chip brazed into a ceramic package. Note the faint image of the devices on the silicon chip and the tiny wires connecting the chip to the ceramic package. Cutaway of an ISM mainframe computer thermal conduction module, showing silicon chips on a hybrid IC package. Helium is circulated through the metal housing to carry away heat produced by the ICs.
A version of this IBM module was about three and one-half inches square and one-fifth of an inch thick. It contained 33 layers, about , metal-filled holes between layers, feet of internal metal wiring, and brazed-on pins for external connections. The IBM module contained over , separate electrical circuits in all and generated so much heat that it required an elaborate cooling system.
This hybrid package, plus the silicon chips, replaced a whole room full of equipment required to do just a fraction of the same computations 20 years earlier. These technologies have profoundly affected our lives, in everything from our personal computers to t h e mainframe computers t h a t handle and the transactions Of banks, Internet, As important as ICs and hybrid Figure 6- IBM mainframe computer IC package.
The magic of ceramics [electronic resource] in SearchWorks catalog
Bottom and top view of IC package, showing the pins for electrical connections and the logic and array silicon chips. The package and chips fit into the ISM thermal conduction module. Ceramic Electrical Insulators Ceramics and glass have been used as electrical insulators since the discovery of electricity. Glass and porcelain were commonly used during the s, and-as you learned in Chapter 2-alumina became important by the mid s for spark plugs in motor vehicles and airplanes.
Since then, these and other ceramics have been used as electrical insulators in just about every device that operates on electricity. One of the oldest uses of ceramic insulators is to insulate power lines that transport electricity from the hydroelectric dam or power-generation plant to our homes. Next time you drive through the countryside, take a closer look at the high Jowers that support the high-voltage electricaltransmission wires. The wires never touch the towers but instead are attached to large ceramic insulators. These ceramic insulators must be able to withstand very high voltage and also must be strong enough to hold the weight of the wires, even during a heavy wind or snow storm.
Once the electricity reaches our home, ceramic insulators line electrical outlets Figure Medium sized electrical insulators of aluminurn oxide. Another important use of ceramic insulators is for substrates or mounting brackets to which other electrical components are attached. The plastic reinforced with glass fibers shown earlier, in Figure , is an example of a substrate, and many of the small parts shown in Figure are ceramic mounting brackets. As mentioned before, ceramics are often used because they can carry heat away more rapidly than plastic can.
Alumina works fairly well and is used, for example, to mount all of the electronics systems in our automobiles. Some products, such as some laser parts, require ceramic materials that carry away heat even better t h a n alumina can.
The Magic of Ceramics
Beryllium oxide and aluminum nitride both conduct heat away at least four Figure Diamond substrates in copper frames. Diamond and copper are superior heat conductors and effectively carry heat away from hightimes faster than alumina-nearly as fast power silicon chip integrated circuits. But the champion is diamond: Diamond carries away heat five to ten times better than do the best metals. Dielectric Ceramics and the Capacitor Ceramic capacitors are electrical devices required in virtually every piece of electrical equipment.
More than one billion are produced each day. The capacitor is actually a special type of electrical insulator called a dielectric. This movement of electrical charge to opposite sides poles of the ceramic is called polarization, and is illustrated in Figure It allows the ceramic to store electricity, which is one of the important tasks of a capacitor. A capacitor is made up of alternating layers of ceramic and metal.
When electricity is passed through the metal layers, the ceramic becomes polarized. Some ceramics work better than others. Schematic illustrating the shift in distribution of electrical charge in a dielectric ceramic during polarization. Note that the positive charges move toward the negative electrode and the negative charges move toward the positive electrode. In fact, barium titanate has played an important role in miniaturization. The radio is a good example.
A typical transistor pocket radio in the s contained around 10 capacitors made with barium titanate. Each of these capacitors was around threeeighths of a n i n c h i n diameter. In , the best dielectric ceramic for capacitors had only o n e five-hundredth t h e polarization of barium titanate. A capacitor made with this material would be much too large to fit into a portable radio.
Capacitors can d o other tasks besides storing electricity. Some can block direct current and allow alternating current to pass; others allow some frequencies of electromagnetic signals-such as radio waves-to pass, and block others. Take a look at the dial of your radio. The numbers you see represent different frequencies of radio waves. New radios use even better dielectric ceramics. Cellular Phones Another important use for dielectric ceramics is in cellular phones, where they act as a bZock filter.
A cellular phone receiver is constantly bombarded by unwanted signals radio waves, TV waves, and so forth , as well as the telephone signals we want Figure 8. Multilayer ceramic capacitors are fabricated similarly to multilayer t o receive. Each of the signals, packages. Layers of dielectric ceramic tape are coated wanted or not, has a different with a thin layer of conductive metal and stacked. The whole assembly is fired to produce a miniaturized wavelength and frequency just as Capacitor. The first successful block filter was over 8 inches long, definitely too big Figure 6- The role of the ceramic block filter in a cellular-phone.
The cellular phone antenna picks up multiple signals from many wireless communication devices. The ceramic block filter selects the desired signal by preventing undesired signals from entering the receiver of the phone. Block filters only became small enough to use in cell phones in the s, but cellular phones are everywhere now. Comparison of an early block filter top, ductors sometime since the first made mostly of metal with later miniaturized ceramic filters. Photo courtesy of Motorola Component Products one was discovered, in The block Croup, Albuquerque, NM. The new ceramics offered new hope for a large reduction in electrical loss, or wastage, during transmission and storage of electricity; for low-cost nuclear magnetic resonance NMR medical scanners affordable to more people for the diagnosis of cancer and other medical problems; for high-speed trains that could ride on air, levitated by magnets; for highly efficient motors; and for incredibly fast supercomputers.
A superconductor has absolutely no resistance to the flow of an electrical current. As we discussed earlier, most materials-even the best metallic conductors, copper Ceramics and the Electronics Age e and silver-have some resistance to the flow of electricity. The electricity has to work a little to get through these metals, which wastes some of the electrical energy and also produces heat. Electrical resistance is like friction. Tie a string to a small block of wood. Pull the block of wood across your cement driveway or a piece of sandpaper.
Now pull the block of wood across a sheet of very smooth glass. It pulls much more easily, because the friction is greatly reduced. This is like the flow of electricity through copper or silver. The resistance is greatly reduced, but still there. This would be comparable to the flow of electricity through a superconductor, with absolutely no resistance or loss or heating. Ceramic superconductor wire and experimental electrical transmission line. Materials become superconductors only at a very cold temperature. The only way to reach this incredibly cold temperature was to dunk the mercury in liquid helium, which is very difficult and expensive t o produce and use.
Liquid nitrogen can be prepared easily and sold at low cost. A tremendous amount of research and development has been conducted on ceramic superconductors since As shown in Figure , experimental electrical-transmission line has been made with this ribbon-shaped wire. Superconductive ceramics also have been built into experimental motors, antennas, microwave devices, and magnetic shielding. Magnetism was first observed many centuries ago in the naturally occurring ceramic material magnetite.
These scientists learned that electrons travel in pairs in orbits around the nucleus of the atom.
SearchWorks Catalog
The two electrons in each pair are spinning, similarly to the way the Earth rotates about its axis. This spin gives each electron a magnetic characteristic, a magnetic moment. However, the two electrons in an orbit spin in opposite directions, so that the magnetic moments are in opposite directions and cancel each other. The primary chemical elements with these unpaired electrons are iron, cobalt, nickel, and manganese. As a result, some metals and ceramics containing these atoms are magnetic, like magnetite, which is made up of iron and oxygen atoms. The modern age of ceramic magnets began in , when J.
Snoeck of Philips Laboratories in Holland synthesized ceramics with strong magnetic properties. He made these magnets similarly to the way that aluminum Ceramics and the Electronics Age h Figure The magnetic field between the magnets is strong enough to suspend one in midair. All of the little magnetic moments were pointing in every possible direction, mostly canceling each other. The secret found by Snoeck was to place the polycrystalline ceramic between two very strong magnets, which forced most of the tiny magnetic moments to line up in one direction, just like when you hold a magnet under a piece of paper with iron filings sprinkled on top: The general term used for a ceramic magnet is fewite.
Once some ceramic magnets have been magnetized, they retain their magnetism extremely well. These are referred to as permanent magnets or hard fewites. Other ceramic magnets can be magnetized and demagnetized readily by changing the direction of an applied magnetic field. These soft fewites are important in television, radio, touch-tone telephones, communications, electronicignition systems, fluorescent lighting, high-frequency welding, transformer cores, and high-speed tape and disk recording heads. Approximately million pounds Each little ring had three copper wires going through the hole in the middle.
The flow of electricity through the wires was controlled so that each magnet could be independently programmed with the positive pole in either one direction or the opposite direction, which allowed storage of one piece of information. You can imagine how large and complex these early computers were. Tiny ceramic magnets used in early computers, some shown on a dime for size comparison. Ceramics will have an even bigger effect i n the future.
Computer capabilities are doubling every 18 months, as scientists and engineers learn how to make smaller and smaller integrated circuits and other electrical devices. We have amazing things to look forward to in the future, made possible by advanced electronics: We left one of the most fascinating electrical characteristics of ceramics out of this chapter: Piezoelectricity defines the magical ability of some ceramics to convert back and forth between pressuremovement or vibration-and electricity.
This unique property is playing an important role in allowing us to interact directly with machines and the world around us. The next chapter is dedicated to all of the incredible ways piezoelectric ceramics are already working for us. If you apply a pressure to a Mot--', tran- piezoelectric ceramic, even as slight as by breathing on renfQS a ancers it or talking to it, the ceramic will give off an electrical -I pulse.
This behavior is used in microphones, underwater sound-detection hydrophones, and pressure- and vibration-detecting sensors. This reaction makes possible the quartz watch, the ultrasonic cleaner, medical ultrasound imaging, and an enormous number of other important products. Probably the most familiar to you are quartz and tourmaline. Quartz also has been an important semiprecious gemstone throughout history, in the form of purple amethyst. Tourmaline also is an important gemstone, usually occurring in shades of red, pink, and green. The phenomenon of piezoelectricity was probably first observed by some Stone Age hunter making a stone axe or spear point, when he struck a piece of quartz with another stone and saw a spark.
The first written description, however, was by Pierre and Jacques Curie, in They studied natural crystals of quartz and tourmaline and other crystals they grew in the laboratory from water-soluble salts. One was sugar a n d t h e o t h e r was called Rochelle salt a n d h a d especially strong piezoelectric behavior.
You can easily grow small crystals of sugar at home. Simply stir a couple teaspoons of sugar into a couple tablespoons of water. The sugar grains will get smaller and smaller and disappear, but the sugar is still present, dissolved in the water. Variety of piezoelectric parts and assemblies. The water will evaporate, and sugar crystals will form on the bottom of the dish. For example, scientists learned that differentsized slices of quartz vibrated when exposed to different frequencies of radio waves, which led to an improved way to tune radios to different broadcast channels.
A little later, piezoelectric Damping cloth crystals were used in phonographs. By , thin slices of Rochelle salt were used in microphones. The piezoelectric ceramic was attached to a diaphragm, like the top of a drum, but much smaller.
When a person spoke into the microphone, the sound waves from his or her voice caused the diaphragm to vibrate at the same wavelength and frequency as the sound waves, much the way your ear drum vibrates to the sound waves hitting it. Schematic of a piezoelectric microphone. Reprinted with sounds and thus fewer vibrations per second.
You can feel that the vibrations are smaller for the high-pitched hum. The diaphragm in a microphone amplifies the vibrations from the sounds entering the microphone and transmits them to the piezoelectric ceramic. Each combination of sounds produces a unique pattern of vibrations that push against the ceramic. The piezoelectric ceramic converts these tiny pressures into a unique electrical signal that matches the unique pattern of vibrations.
All of the early piezoelectric materials were single crystals. Gray obtained a patent on a piezoelectric polycrystalline ceramic called barium titanate made up of atoms of barium, titanium, and oxygen. This was a major breakthrough as the polycrystalline ceramic was stronger and more durable than single crystals, cheaper to make, and not soluble in water like Rochelle salt. An early use of barium titanate was for fish finders. Improved polycrystalline piezoelectric ceramics were developed during the Piezo Power Qh s, culminating in the invention, in , of Zead zirconate titanate PZT by Bernard Jaffe.
This material, made up of atoms of lead, zirconium, titanium, and oxygen, had especially strong piezoelectric behavior and opened the door for hundreds of new applications. As scientists and engineers became more familiar with piezoelectric ceramics, they found a wide range of imaginative ways to use these remarkable materials. As with the microphone, they could use piezoelectric ceramics to detect vibrations, even those as minute as sound waves.
They could apply an alternating current, such as the electricity you get every day when you plug in your TV or toaster, to a piezoelectric ceramic to make it vibrate. If they applied a direct current, such as from a battery, they obtained a controlled amount of mechanical deflection movement, shape change of the ceramic. The amount of deflection could be controlled from millionths of an inch to tenths of an inch by the amount of voltage applied, the thickness of the piezoelectric ceramic, and the number of layers of ceramic that could be built into a stack. They observed that sometimes the electrical input forced the piezoelectric ceramic to vibrate at its natural frequency like a rubber band vibrates when you pluck it and either emit sound, like the vibrating string of a guitar, or create movement that could be used as a measurement of time, like in a quartz watch.
They learned ways to apply a controlled amount of pressure or a sharp blow to the piezoelectric ceramic and produce a high-voltage spark that could ignite a fuel, as an alternative to a spark plug. The rest of this chapter presents many different uses for piezoelectric ceramics that rely on these different ways piezoelectricity works. Hydrophones An early use for piezoelectrics was in hydrophones, which were important during the Second World War for detecting enemy submarines and monitoring other ships from submarines.
Remember the old submarine movies? A submarine would have all its engines off, and the crew would be listening electronically for sounds made by other submarines or ships. Hydrophones were the devices that made this clandestine listening possible. Sound waves from the engine of another submarine or a surface ship would travel through t h e water and strike the hydrophone.
The piezoelectric crystals detected the pressure of these U sound waves and gave off electrical sigFigure Schematic of a hydrophone.
Account Options
It was developed to detect, as well as to determine the distance of, underwater objects such as another submarine, even with its engines off, or the hull of a surface ship. Sonar can be compared to the navigation technique of a bat or to the radar gun used by police to catch speeding motorists. Certain types of waves sent out from a Piezo Po wer Kx device travel through the air in the case of the bat or radar gun or water in the case of sonar until they hit a solid object. Some of these waves bounce off the object and come back, to be detected by the sender.
In the case of sonar, sound waves are emitted. A sound wave is produced by applying a pulsed electrical input to a piece of piezoelectric ceramic. Each electrical pulse causes the ceramic piece to distort, or bend, which produces a sound acoustic wave in the water. A piezoelectric ceramic used in this way is called a transducer. Each wave spreads out and travels through the water, just like a wave spreads out and travels away from a pebble that you drop into a pond.
When the waves run into a solid object, they reverse direction and head back to the transducer. Now the transducer acts like a hydrophone and converts the pressure from the waves back into electrical pulses. Sonar works as the eyes of the submarine, just as a pulse-echo system replaces the eyes of a bat in total darkness. Decoys Because sonar gives us a way to detect and track both underwater and surface ships, it has great military value.
Of course, every military device seems to have a counter-device designed to defeat it. How do you defeat a piezoelectric device? With another piezoelectric device. Pingers can be released by a submarine or ship to act as decoys to send false signals to enemy ships. Called a doppler sonar velocity s y s t e m a n d mounted o n the bottom of the ship, this device sends ultrasonic vibrations to the bott o m of t h e body of water a n d uses t h e reflections to calculate the speed of the boat. It can even correct for ocean currents.
Doppler sonar velocity system. Measuring Accurately measuring approximately 6 inches in diameter, the system can measure the speed of a ship to a precision of 0. The piezoelectric device is a big improvement over the previous system: Fish Finders Another category of piezoelectric ceramic devices that operates in the same fashion as sonar is used for finding and tracking schools of fish. Fish finders have been in use since the late s and have dramatically increased the efficiency of the world fishing industry, unfortunately even resulting in overfishing in some areas.
How do ceramics improve your everyday life? It describes how the ceramic material functions, and why it is superior to other materials, while teaching key scientific concepts like atomic structure, color, and the electromagnetic spectrum. The Best Books of Check out the top books of the year on our page Best Books of Product details Format Hardback pages Dimensions Looking for beautiful books? Visit our Beautiful Books page and find lovely books for kids, photography lovers and more. Table of contents Our Constant Companions. From Pottery to the Space Shuttle.
The Beauty of Ceramics. Amazing Strength and Stability. Ceramics and the Electronics Age. Ceramics and the Modern Automobile. The Hardest Materials in the Universe. Energy and Pollution Control.